Poly(ADP-ribose) polymerase (PARP) inhibitors (PARPis) are promising cancer treatments because they block a cell’s ability to repair DNA damage. Four PARPis are currently approved for use in the clinic, and more than 200 others are making their way through (pre)clinical phases.1 However, the ability of these anticancer therapies to effectively and specifically eliminate malignant cells depends on several factors that scientists should keep in mind during the screening and drug development process.
Maintaining genomic integrity is critical for cell survival. For this reason, at least 150 different proteins form an intricate DNA damage response (DDR) network that constantly scans and repairs a cell’s genome.2 PARP1 is one of the first responders to damaged DNA, and its importance is reflected in its abundance: this enzyme is one of the most common nuclear proteins.3 When PARP1 binds damaged DNA, it adds poly(ADP ribose) (PAR) chains to its own protein backbone and to other DDR proteins, which recruits and activates them.4 PARylated PARP1 next detaches from the DNA so that the other PARylated proteins can initiate the repair process.
As is the case for many proteins that perform essential functions such as safeguarding a cell’s genetic material, there are several PARP proteins that functionally overlap to a certain extent. The PARP protein family comprises 17 members, but PARP1 and PARP2 are the main proteins involved in DNA repair. Both proteins regulate the DDR network, but PARP1 also regulates transcription and induces apoptosis when DNA is damaged beyond repair. In contrast, PARP2 also has regulatory functions in epigenetic, proliferative, and inflammatory processes and is important for spermatogonia, thymus, and adipose tissue development.1,5
Most tumor cells exploit weaknesses in the DNA repair system to induce genomic instability and accumulate genetic changes that promote survival or metastasis. For example, BRCA1 and BRCA2 mutations impair a cell’s ability to repair double-stranded DNA breaks through homologous recombination (HR), which increases an individual’s susceptibility to breast, ovarian, or prostate cancer.1,6 However, the loss of HR-dependent DNA repair means that these tumor cells rely on other repair pathways for survival, exposing their therapeutic Achilles heel to scientists.
When scientists developed PARPis, which specifically block these survival DNA repair pathways by inhibiting PARP protein function, they efficiently targeted HR-deficient cancer cells for apoptosis through a process called synthetic lethality.1,3 Follow-up studies showed that PARPis also inhibit DNA repair in response to chemotherapies that induce single-stranded DNA breaks, paving the way for this therapeutic class to treat various cancer types.
Specificity is a key determining factor of PARPis’ clinical safety and efficacy profile. Current FDA-approved drugs target both PARP1 and PARP2, but they also inhibit other PARP family members and, therefore, increase unwanted side effects.7 To reduce PARPis’ off-target activity and minimize clinical side effects, researchers screen for molecules that specifically target PARP1/2.
Currently approved drugs reduce PARP1/2’s catalytic activity to a similar extent, but their cytotoxic effects depend primarily on how efficiently they trap PARP1 on damaged DNA.3,8 PARPis compete with NAD+ for binding PARP1/2’s catalytic site. Without NAD+, PARPs fail to self-activate and remain bound to the damaged DNA, shielding it from other DDR proteins.7 Scientists recently found that trapping PARP1, but not PARP2, to DNA with PARPis resulted in increased cytotoxicity towards cancer cells.1,7,8 Therefore, PARPis drug screens should include assays that quantify PARP-trapping ability and distinguish an inhibitor’s selectivity to PARP1 or PARP2.
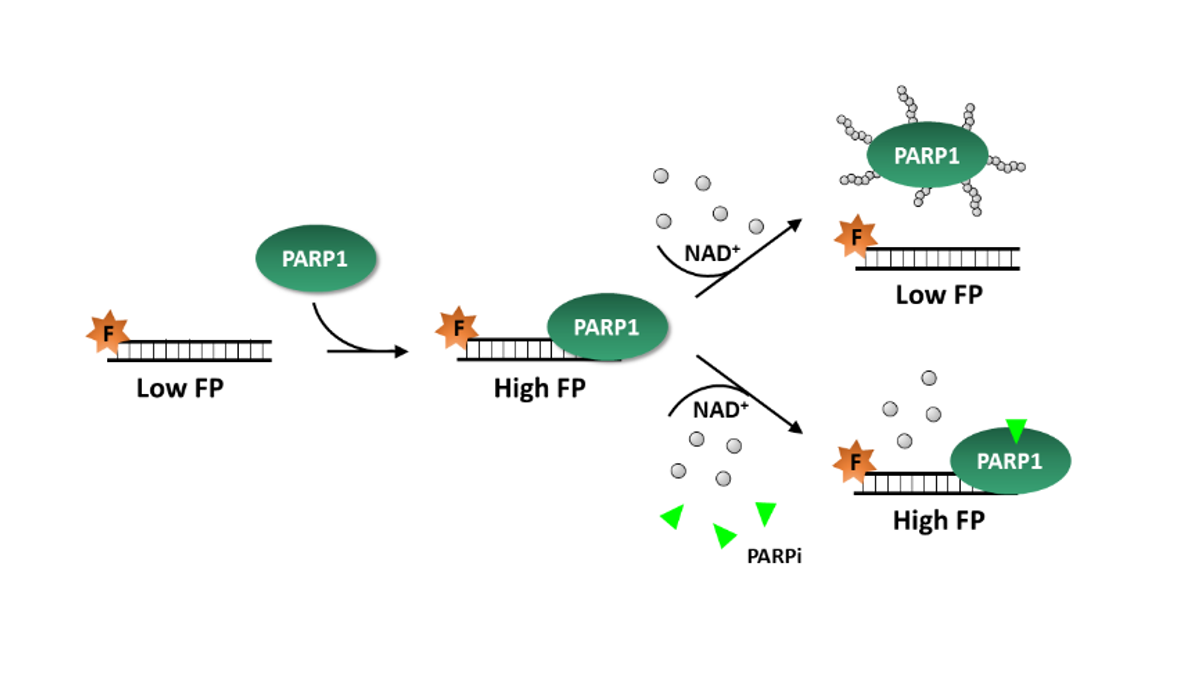
Most commercially-available PARP activity assays quantify PARylation of target proteins, such as histones, and test only one PARP enzyme at a time. In contrast, BPS Bioscience’s PARPtrap™ Combo Assay Kit for PARP1 and PARP2 compares a molecule’s ability to trap PARP1 versus PARP2 in the same assay. The assay uses fluorescently-labeled DNA probes that emit polarized light depending on PARP1 or PARP2 binding. These probes have high fluorescence polarization (FP) when PARP1 or PARP2 is bound to DNA. When scientists add NAD+ to the assay, PARylated enzymes detach from the probe, reducing FP levels. If, instead, they add NAD+ and a PARPi, the inhibitor’s trapping ability increases FP in a dose-dependent manner. Because PARPtrap™ comes in convenient 96- or 384-well plates and includes all of the necessary reagents, researchers can incorporate it in high-throughput drug discovery screens for molecules that enhance PARP1 or PARP2 trapping on DNA. This way, the PARPtrap™ assay allows researchers to efficiently screen their libraries for the most specific and effective PARPis.
References
- M. Rose et al., “PARP inhibitors: clinical relevance, mechanisms of action and tumor resistance,” Front. Cell Dev. Biol, 8:564601, 2020.
- R.D. Wood et al., "Human DNA repair genes,” Science, 291, 1284–9, 2001.
- J. Rudolph et al., “Inhibitors of PARP: Number crunching and structure gazing,” PNAS, 119(11): e2121979119, 2022
- D. Slade, S. Eustermann, “Tuning drug binding,” Science, 368(6486):30–1, 2020.
- S.O. Ali et al., “Understanding specific functions of PARP-2: new lessons for cancer therapy,” Am J Cancer Res, 6(9): 1842–63, 2016.
- C.J. Lord, A. Ashworth, “BRCAness revisited,” Nat Rev Cancer, 16(2):110-20, 2016.
- D. Slade, “PARP and PARG inhibitors in cancer treatment,” Genes Dev, 34(5-6): 360–94, 2020.
- Y. Pommier et al., “Laying a trap to kill cancer cells: PARP inhibitors and their mechanisms of action,” Sci Transl Med, 8(368):368er7, 2016.