ABOVE: Fingerprint patterns are laid down in a set of waves, starting and spreading from distinct anatomical sites. The image illustrates three separate patterning waves (green, pink, and blue) converging to form a loop pattern. JAMES GLOVER
How the unique arrays of swirls, arches, and loops on the tips of our fingers form is a longstanding scientific enigma. Now, a paper published February 9 in Cell has solved the mystery, revealing not only the process by which fingerprints are formed, but also the genes responsible. And, it turns out, our distinctive prints stem from the same phenomenon that gives zebras their stripes and leopards their spots.
For several years, University of Edinburgh developmental biologists Denis Headon, James Glover, and their colleagues had been investigating how skin develops and matures, with a special interest in fingerprints. Fingerprints form before birth and may have evolved because they improve our ability to grip onto or feel the texture of objects. Still, scientists have long been stumped when it comes to the actual mechanisms by which these distinctive patterns develop. Understanding this process could help improve therapies for human congenital conditions like ectodermal dysplasias, Headon says, or even lead to better ways of regenerating skin.
It had been previously suggested fingerprints may arise from some form of pre-existing template, the way finger skin cells communicate, or even just simple wrinkling of the skin. To figure out which, if any, of these ideas is correct, Headon and his team used a variety of methods, including examining mouse and human tissue under a microscope, looking at the gene expression of single cells using single nucleus RNA sequencing, growing groups of cells on culture plates, and computer modeling.
“One thing that stood out to me was just the breadth of different approaches that they use in the paper,” Jeff Rasmussen, a developmental biologist at the University of Washington who was not involved in the work, tells The Scientist. “All of those different types of methodology really help them build [a] cohesive story.”
That story begins in the outer layer of body tissue, called the epithelium. Headon’s team ultimately found that fingerprints start out looking very similar to hair follicles: Both begin as small discs of cells on the epithelium, and in both cases, the cells turn on genes for a suite of proteins including EDAR and WNT—which are respectively related to how epithelial cells and cells in general migrate, differentiate, and mature. However, hair follicles go on to recruit cells from layers below the epithelium, forming a deep tube where hair will eventually grow. Slight differences in gene expression prevent this recruitment step from happening in fingerprints.
Those same differences in gene expression also seem to set up a Turing pattern, named for the English mathematician Alan Turing who first hypothesized its existence. Back in 1952, Turing suggested that natural biological patterns like stripes or spots could form in the presence of two molecules: a slow-moving activator and a fast-moving inhibitor. The activator would do three things: 1) tell cells to do something, such as make colored pigment; 2) tell cells to make more activator; and 3) tell cells to start making its inhibitor. Meanwhile, the inhibitor tells the cell to slow down activator production (and thus, ultimately, to make less of itself). This means that the activator and inhibitor are always made in overall proportion to each other, and the whole system can propagate from even a single initiation point.
The process may be admittedly a bit hard to picture, but after a while, this pattern of activation and inhibition can result in a series of stripes or spots depending on how the effect ripples outward from the starting point. In the new study, the researchers found that the WNT and EDAR proteins act as activators that create ridges in the forming skin, while proteins called BMPs (which are produced in response to WNT) act as inhibitors.
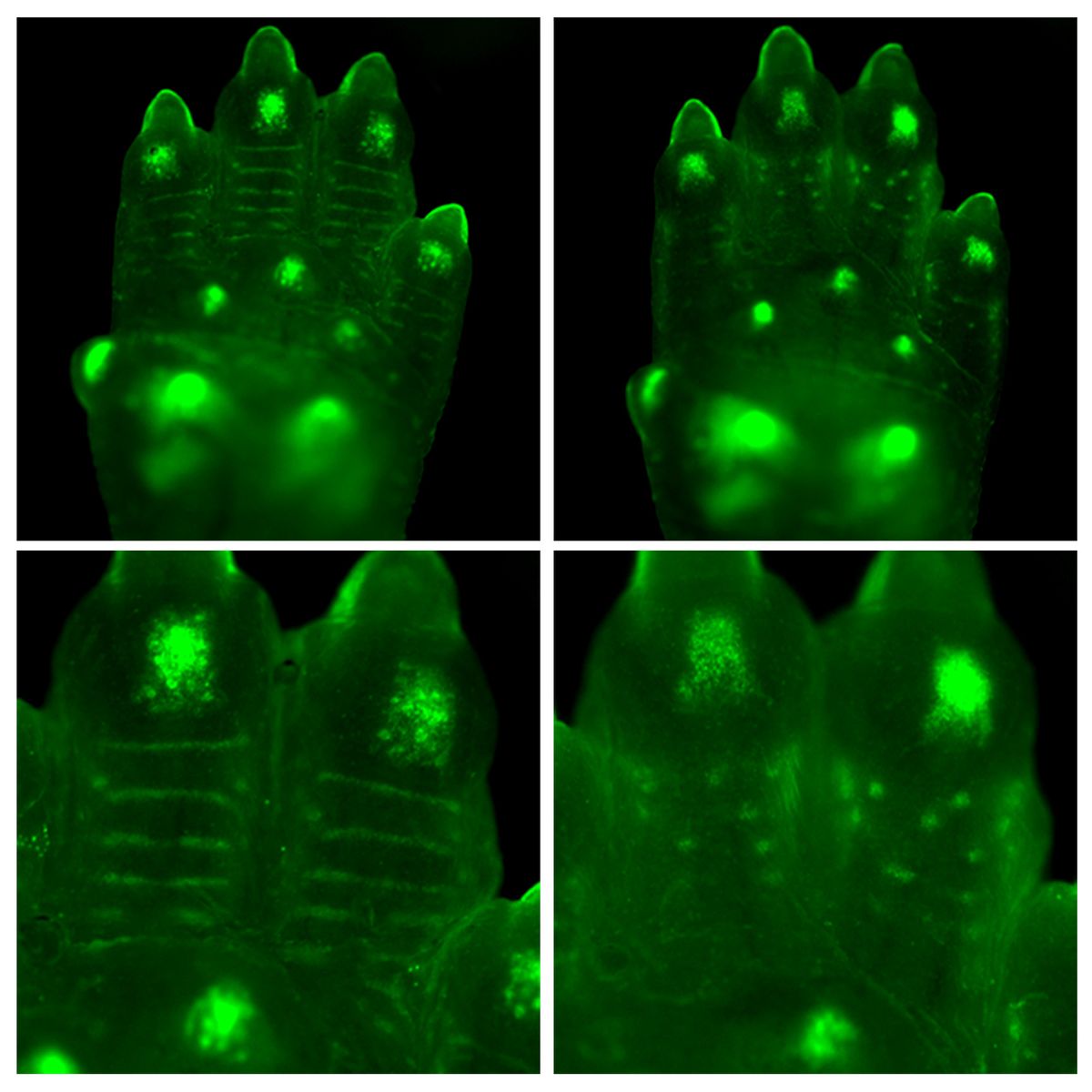
Today, we know that Turing patterns are responsible for things like zebra stripes and leopard spots, perhaps even the arrangement of fingers on our hands. Analogous phenomena in physics can even explain patterns like the stripes in sand dunes. In their paper, Headon and colleagues saw the phenomenon play out in the fingertips of mice with mutations to the gene for EDAR, when the stripey ridges of their fingerprints turned into spotty bumps—something difficult to explain with anything other than a Turing pattern, according to study author James Glover.
The pattern seems to originally start in three areas in humans: up near the nail, towards the center of the fingertip, and down near the crease from the first knuckle. As the Turing pattern matures, the fingerprint ridges then spread out as a series of waves from these initiation sites, eventually meeting in the middle and forming the unique fingerprint pattern each of us is born with.
“It’s the way that this patterning system is switched on in different locations and oriented in different locations . . . that’s what determines fingerprint type,” says Headon.
Some of the team’s colleagues—Benjamin Walker, Adam Townsend, and Andrew Krause—created an online simulator called VisualPDE where folks can experiment with Turing patterns and initiation sites. VisualPDE’s simulation is not unique to fingerprints but can illustrate how small changes can create unique patterns.
Rasmussen says he’d be interested in seeing if scientists could reprogram the process, creating hair follicles or prints where there had been none before. That’s the hope, Headon says: that somewhere down the road this work could lead to therapies for congenital conditions or medical regeneration.
But there’s also value in learning more about the many ways Turing’s patterns show up and connect life on Earth, and the subtle ways they can lead to such a wide variety of forms, says Glover. “From this, you can . . . gain a broader understanding of how patterns form in biology,” he explains. “These systems do vary between organs and species. So chipping away at looking at the different mechanisms, different systems will be really useful going forward.”